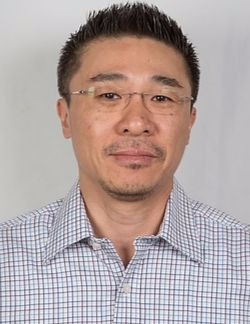
Department
School
Expertise
Research Interests
Using the budding yeast, S. cerevisiae, as a model system, my laboratory studies several areas of research related to genome stability and plasticity: (1) Elucidating the mechanism(s) responsible for integrating nutrient signals with chromosome segregation and the DNA damage response (DDR), (2) Mapping complex haploinsufficient interactions that lead to chromosome instability, (3) Investigating how genome instability is linked to the mechanism of neurodegeneration in Huntington's disease, and (4) Developing single cell technologies to study the aging process. We apply and develop genome-scale and single cell resolution tools with the aim of building a comprehensive understanding of metabolic signaling and its connection to chromosome structure and function, particularly within the context of genome maintenance and the etiology of disease.
(1) Nutrient availability is an important factor in tumorigenesis and in maintenance of healthy tissue. Notably, cancer cells are known to harbor mutations in genes that serve important roles in nutrient signaling and as early as the 1930’s Otto Warburg provided evidence that cancers have a unique metabolic program that differs profoundly from normal cells. Key nutrients such as glucose, amino acids, phosphate, and ammonium can activate signaling cascades resulting in significant changes in the transcriptome that modulate cellular physiology and the decision to enter or exit the cell division cycle. The Ras/Protein kinase A (PKA) signaling pathway is a key regulator that senses intra- and extracellular glucose and transduces signals to activate or inhibit cell growth in both yeast and human cells. We previously discovered that cells with deregulated PKA activity has greater than a 20-fold increase in the rate of chromosome loss compared to wild-type cells. We are currently using targeted and genome-scale genetics and molecular approaches to elucidate the mechanism by which PKA signaling can modulate chromosome segregation fidelity. We also have a very limited understanding of how metabolic signaling impacts the DDR, which ultimately can lead to changes in the integrity of the genome. In particular, glucose deprivation can occur when blood supply to cells become restricted as is the case in tissue ischemia and in solid tumors, where rapidly proliferating cells compete for limited glucose and oxygen. Toward investigating how metabolic signaling is integrated with the DDR we are (1) using genome-scale genetics in the model system, S. cerevisiae, to explore if different nutrient conditions lead to “rewiring” of the network of pathways required for a proper DDR and (2) dissecting the changes in protein-protein interactions essential for the DDR under specific nutrient conditions.
(2) Copy number variation (CNV) and aneuploidy are common features of cancer. In particular, recurrent hemizygous focal deletions are observed in several cancer types and the vast majority does not harbor tumor suppressor genes. This raises the possibility that these regions carry haploinsufficient genes that contribute to tumorigenesis. In addition, many of the same cancers are also aneuploid, yet the mechanism of aneuploid formation is unclear. This motivates the following question: Might there be haploinsufficient genes that predispose cells to chromosome missegregation leading to aneuploidy? Toward this end, we developed a method to perform genome-wide screens to identify haploinsufficient mutations that cause chromosome instability in S. cerevisiae. The genes revealed from this work point to a variety of biological processes that impact genome stability. Notably, this work uncovered a new link between metabolic signaling and chromosome stability and a novel function for gamma-tubulin in controlling spindle assembly during chromosome segregation. Based on this work, we are now (1) investigating mechanisms of how altered gene dosage of metabolic signaling genes can cause chromosome instability and (2) constructing a comprehensive haploinsufficiency genetic map of metabolic pathways used to maintain genome stability by studying epistasis of haploinsufficient mutants.
(3) Huntington's disease is the most frequently inherited neurodegenerative disease that is associated with the loss of cortical and striatal neurons that are found in the brain. Typically individuals show symptoms such as a loss in motor coordination, and higher order cognitive abilities such as reasoining and thinking, which begin between the ages of 30-50, and progressively worsen over the course of 10-25 years. Presently there is no cure, however, it is well established that an expanded glutamine repeat in the huntingtin (HTT) gene is known to cause the disease. The mutant HTT protein is known to form aggregates in human neurons and it is thought that the aggregates lead to neuronal cell death, yet we still lack a clear mechanism of how these aggregates function. Expression of the first exon of HTT with the expanded glutamine repeats are also known to form aggregates in yeast and also causes a loss in viability. The mutant HTT aggregates are thought to cause protein stress and several groups have mapped out a large number of genetic interactions suggesting that HTT aggregates affect numerous processes, including the formation of reactive oxygen species. We have found that mutant HTT modulates genome stability and working to understand the mechanism of action. We are also investigating similar possibilities with other neurodegenerative diseases that are associated with proteinopathies.
(4) Aging has been described as the greatest carcinogen. It is well established that as cells age, the frequency of mutation increases. In yeast, it is known that nearing the last quarter of their life span there is a significant increase in loss of heterozygosity, demonstrating that as part of the aging process the genome becomes less stable. The mechanisms are not yet fully established and we still do not have a clear understanding of all the physiological changes that occur as a result of aging. My laboratory in collaboration with Dr. Xiaolong Luo in the Department of Mechanical Engineering at Catholic University are developing microfluidic platforms coupled with fluorescence microscopy to allow us to (1) investigate the changes in cell cycle kinetics and (2) monitor changes in the DDR, all as a function of age and at single cell resolution.